Prediction of radiosensitivity in primary central nervous system germ cell tumors using dynamic contrast-enhanced magnetic resonance imaging
Introduction
Primary central nervous system (CNS) germ cell tumors (GCTs) are rare and account for 0.5% of all primary CNS tumors in the West (1), while they are more common in China and other Asian countries and constitute approximately 2.0-7.9% of primary CNS tumors in adults or children (2,3). GCTs encompass a wide pathologic spectrum, and each specific subtype has different treatment options and prognosis. However, there are some overlaps in clinical presentation and tumor markers among GCTs subtypes, and conventional neuroimaging characteristics are of little diagnostic value for differentiating GCT subtypes. Furthermore biopsy is not widely practiced recently, because anatomically GCTs are located deep and adjacent to some important structures and vessels. In addition the sample is often insufficient to confirm the diagnosis (4). Thus, diagnostic radiation serves as an alternative method to determine the histological type of GCTs (5,6). But side effects and late sequelae induced by radiation therapy (RT) have led to questions on the reliability of diagnostic radiation. Hence we tried to find a non-invasive imaging method to replace the diagnostic radiation for predicting tumor response to RT, so that the treatment strategy could be determined or modified according to radiosensitivity of tumors, rather than just based on histological assessment.
Dynamic contrast-enhanced magnetic resonance imaging (DCE-MRI) is a non-invasive quantitative technique in assessing microvascular permeability, and in distinguishing benign from malignant tumors of the brain, breast, kidney, and sino-nasal (7-9). Recently several studies demonstrated that DCE-MRI data could monitor the early changes in tumor response to therapy, so that it can be used to evaluate tumor response to various treatments before the tumor size appears to change (10-13). Furthermore kinetic parameters derived from DCE-MRI can reflect tumor microenvironment variables, which play an important role in the assessment of tumor response to RT (14). Certain studies evaluated the predictive role of DCE-MRI in assessing tumor response to radiotherapy carried out for the cervix, colorectal, and brain tumors (15-17). However, to date clinical research regarding GCTs’ response to RT is hardly seen. Therefore, our study aims to investigate the changes in pharmacokinetic DCE-MRI parameters in tumor tissue before RT and evaluate the feasibility of DCE-MRI in predicting radiosensitivity of GCTs.
Materials and methods
Patients
The study was approved by the institutional review board of Beijing Tiantan Hospital and informed consent was obtained from all patients. Thirty-five patients clinically diagnosed with primary CNS GCTs from Jun 2013 to Nov 2014 were recruited in this study. Patients were included if they fulfilled the following inclusion criteria: patients with untreated mass in pineal, basal ganglia, and/or suprasellar region, which were suspiciously diagnosed as GCTs by clinical symptoms, tumor markers, and neuroimaging manifestations; normal or slightly elevated levels of β-human chorionic gonadotropin (β-HCG) in serum and cerebrospinal fluid, and normal levels of α-fetoprotein (AFP). Exclusion criteria were elevated levels of β-HCG, i.e., >1,000 mIU/mL, children younger than 5 years, stereotactic radiotherapy, chemoradiotherapy, or any other MR contraindications.
DCE-MRI was conducted prior to diagnostic radiation. All patients received 10 Gy diagnostic radiation after baseline MRI examination. Two weeks after diagnostic radiation, patients were evaluated on radiosensitivity and divided into two groups: responsive group and non-responsive group. Criteria for responsive groups were reduction in tumor size ≥50% compared with baseline tumor size in MRI (18), and levels of β-HCG, AFP in serum and cerebrospinal fluid within normal limits.
MRI protocol
MRI was performed on a 3.0 T MR system (Trio Tim, Siemens healthcare, Germany) with an eight-channel head coil. The MRI protocol included sagittal T1WI [repetition time (TR)/echo time (TE), 1,200/11 ms; field of view (FOV) 220 mm × 220 mm] and axial T1WI (TR/TE, 2,000/9.8 ms; FOV 220 mm × 186 mm), axial T2WI (TR/TE, 4,500/84 ms; FOV 220 mm × 186 mm), slice thickness/gap, 5.0/0 mm, matrix 256×256, T1-magnetization prepared rapid gradient echo (T1-MPRAGE) (TR/TE, 2,300/3.01 ms; slice thickness/gap, 1.0/0 mm, matrix 256×256, FOV 240 mm × 240 mm).
DCE-MRI processing
DCE-MRI was performed using a T1-weighted 3D radial gradient-recalled echo sequence (Volumetric Interpolated Breath-hold Examination, VIBE). The parameters are as follows: TR/TE: 3.86/1.39 ms, flip angle: 12°, slice thickness/gap: 3.0/0 mm, matrix: 256×256, FOV: 220 mm. The acquisition consisted of 50 measurements with a temporal spacing of 4.0 s. Before the dynamic scan, a multiflip-angle approach was performed for T1 mapping. After the fifth phase of dynamic scan, a bolus of gadolinium-diethylene triamine pentacetate acid (Gd-DTPA) (Bellona, Beijing, China) was injected intravenously at a rate of 4 mL/s, a dose of 0.2 mmol/kg of body weight was administered and followed immediately by a 20 mL continuous saline flush.
DCE-MRI analysis
The DCE-MRI data were sent for post-processing to Tissue 4D software (Siemens Medical Systems). Motion correction and registration were performed before pharmacokinetic evaluation. The artery input function was measured automatically by gadolinium dose in an intermediate mode. The transfer constant (Ktrans), extravascular extracellular space (Ve), and rate constant (Kep) were calculated using the Tofts and Kermode pharmacokinetic model (19). Meanwhile, maps for pharmacokinetic parameters were generated automatically. The region of interest (ROI) was selected by an experienced neuroradiologist inside the tumor as well as in normal brain tissue to obtain the pharmacokinetic DCE-MRI parameters. Normal brain tissue was defined as right or contralateral frontal subcortical white matter which showed no enhancement. During the drawing of ROI, cystic, necrotic, hemorrhagic regions, and normal vessels within the ROIs were avoided. Relative pharmacokinetic DCE-MRI parameters were defined as the ratio of DCE-MRI parameters between tumors and normal brain tissue.
Statistical analysis
Statistical analysis was performed using SPSS 17.0 (SPSS Inc., Chicago, IL, USA). Differences in DCE-MRI parameters between groups were compared by Wilcoxon signed-rank test and Mann-Whitney U-test. The correlation between DCE-MRI parameters and changes in tumor volume was analyzed using Spearman’s correlation analysis. Taking radiosensitivity as a gold standard for reference, the cut-off value of DCE-MRI parameters was calculated by receiver operating characteristic (ROC) curve. The area under the curve (AUC), sensitivity, specificity, positive predictive value (PPV), and negative predictive value (NPV) were used to evaluate diagnostic value of DCE-MRI parameters. P<0.05 was considered statistically significant.
Results
Patient characteristics
Of 35 patients suspected for primary CNS GCTs recruited in our study (mean age, 14.2±5.2 years; age range, 8-28 years), 30 were male and 5 were female. Twelve patients were diagnosed as non-responsive or partly responsive to RT and included in the non-responsive group, and 23 were in the responsive group. The two groups were balanced in terms of age, gender, and initial tumor size (P<0.05).
Comparison of differences in DCE-MRI parameters between tumors and normal brain tissue
Table 1 shows the mean Ktrans, Kep and Ve values of normal brain tissue and tumors. Compared with contralateral normal brain tissue, a significant elevation of Ktrans and Ve as well as a significant reduction in Kep was observed in tumors (P<0.01).

Full table
Relationship between DCE-MRI parameters and changes in tumor volume
There was no significant correlation between DCE-MRI parameters and changes in tumor volume. The relative value of DCE-MRI parameters was not correlated with changes in tumor volume (P>0.05).
Comparison of differences in DCE-MRI parameters between responsive and non-responsive groups
Table 2 shows the mean values of DCE-MRI parameters of both groups. There was a significant elevation of Ktrans, relative Ktrans, and relative Kep in the responsive group (Figures 1,2), whereas no significant difference was found in Kep, Ve, and relative Ve between the two groups.
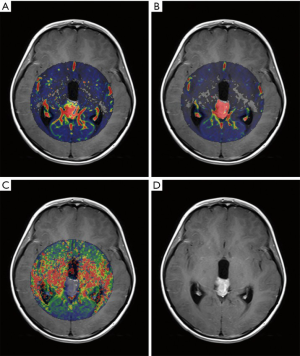
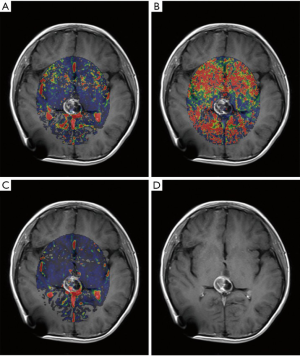
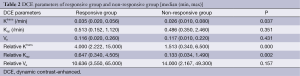
Full table
ROC curve analysis of DCE-MRI parameters for assessment of radiosensitivity in GCTs
To determine the cut-off value of DCE-MRI parameters for predicting radiosensitivity, we analyzed Ktrans, relative Ktrans, and relative Kep, which showed a significant difference between the two groups by ROC curve analysis (Figure 3). ROC curve analysis showed that the cut-off value of Ktrans, relative Ktrans, and relative Kep was 0.022, 2.066 and 0.188, respectively, and indicated a good level of diagnostic accuracy for the prediction of radiosensitivity. The AUC, sensitivity, specificity, PPV, NPV parameters for predicting radiosensitivity are presented in Table 3. As shown in Table 3, relative Ktrans showed best diagnostic value in predicting radiosensitivity with a sensitivity of 100%, specificity of 91.7%, PPV of 95.8%, and NPV of 100%.
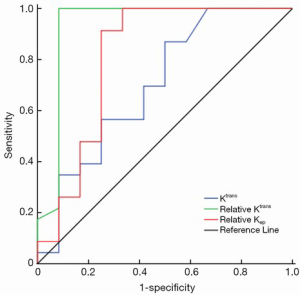

Full table
Discussion
The present study investigates the differences in pharmacokinetic DCE-MRI parameters of normal brain tissue and tumors in patients with CNS GCTs, and demonstrates the efficacy of DCE-MRI in predicting radiosensitivity of CNS GCTs.
Characterized by angiogenesis, solid tumors often have abnormal blood vessel structure, function, and enhanced microvascular permeability. Currently both animal studies (20,21) and clinical studies (7-9) revealed that DCE-MRI is widely used to assess microvascular permeability, which may further contribute to distinguish benign from various solid malignant tumors. However, very little is currently known concerning tumor vasculature of CNS GCTs. In our study, we found that, similar to most solid tumors, tumor tissue of GCTs has a mildly increased Ktrans. It indicates that the increased microvascular permeability of GCTs might be due to the disruption of the blood-brain barrier (BBB). This finding is consistent with previous studies, which proposed that BBB is relevant in patients with metastatic GCTs (22). Furthermore, an elevation of Ve in GCTs corresponds to a relative increase in extravascular extracellular spaces, which implied that GCTs changed in a tumor microenvironment.
Radiosensitivity is an important characteristic of CNS GCTs concerning tumor response to RT. Although optimal management for GCTs remains controversial, RT is well known as a critical component in multi-modal treatment of GCTs and provides favorable outcomes and long-term survival (1,23-25). Thus, prediction of tumor response to RT is a prerequisite for optimal selection of the timing and the field of RT. Kinetic data obtained by DCE-MRI regarding the changes in microvascular permeability and microenvironment can enhance understanding of the underlying mechanism of tumor response to RT (15-17). Although our attempt to analyze the relationship between pharmacokinetic DCE-MRI parameters and changes in tumor volume failed to show any significant correlation, the differences in Ktrans, relative Ktrans, and relative Kep between the responsive and non-responsive groups were significant, which was consistent with the result of Hans’s study (17). They performed DCE-MRI in 31 patients with rectal cancers before chemoradiotherapy, and demonstrated that responsive tumors had higher values of DCE-MRI parameters than non-responsive tumors. This finding indicated that different response of GCTs was caused by pharmacokinetic parameters concerning tumor microvascular permeability.
In addition, we used the cut-off value of Ktrans, relative Ktrans, and relative Kep to stratify GCTs for predicting radiosensitivity. Relative Ktrans showed the best diagnostic value in predicting response with a sensitivity of 100% and specificity of 91.7% by ROC curve analysis. Unlike absolute value, relative pharmacokinetic parameters are more objective and accurate, and can overcome the impact of environment and individual differences. Our study showed that relative Ktrans may be more applicable than absolute value with high sensitivity and specificity. Given methodologic simplicity, noninvasiveness, and wider applicability, we propose that relative Ktrans derived from DCE-MRI should replace diagnostic radiation as a useful and requisite step in the diagnostic procedures.
Our study has some limitations. The response evaluation criteria for diagnostic radiation were inconsistent with the requirement of the Response Evaluation Criteria in Solid Tumors Standard (26). However, previous studies tested the precision and repetition of this diagnostic means using the same criteria (18,23). Moreover, these criteria of diagnostic radiation have been applied in clinical practice for several decades (27). Additionally, owing to low prevalence of GCTs, the sample size of this study is relatively small and the interpretation of the results will be limited.
Conclusions
This study demonstrates that relative Ktrans derived from DCE-MRI is helpful in predicting radiosensitivity of GCTs. It can be used to guide clinical choice of RT and assist in finding new clinical perspective and insight for clinical diagnosis of GCTs. Our results imply that DCE-MRI may replace diagnostic radiation and serve as a novel and reliable approach to assess radiosensitivity of GCTs before treatment and represent a requisite step in the diagnostic procedures.
Acknowledgements
Funding: This study was supported by Beijing Natural Science Foundation (No. 7122029).
Disclosure: The authors declare no conflict of interest.
References
- Thakkar JP, Chew L, Villano JL. Primary CNS germ cell tumors: current epidemiology and update on treatment. Med Oncol 2013;30:496. [PubMed]
- Shibamoto Y. Management of central nervous system germinoma: proposal for a modern strategy. Prog Neurol Surg 2009;23:119-29. [PubMed]
- Zhou D, Zhang Y, Liu H, et al. Epidemiology of nervous system tumors in children: a survey of 1,485 cases in Beijing Tiantan Hospital from 2001 to 2005. Pediatr Neurosurg 2008;44:97-103. [PubMed]
- Shinoda J, Sakai N, Yano H, et al. Prognostic factors and therapeutic problems of primary intracranial choriocarcinoma/germ-cell tumors with high levels of HCG. J Neurooncol 2004;66:225-40. [PubMed]
- Packer RJ, Cohen BH, Cooney K. Intracranial germ cell tumors. Oncologist 2000;5:312-20. [PubMed]
- Echevarría ME, Fangusaro J, Goldman S. Pediatric central nervous system germ cell tumors: a review. Oncologist 2008;13:690-9. [PubMed]
- Huang W, Li X, Morris EA, et al. The magnetic resonance shutter speed discriminates vascular properties of malignant and benign breast tumors in vivo. Proc Natl Acad Sci U S A 2008;105:17943-8. [PubMed]
- Alonzi R, Padhani AR, Allen C. Dynamic contrast enhanced MRI in prostate cancer. Eur J Radiol 2007;63:335-50. [PubMed]
- Xian J, Du H, Wang X, et al. Feasibility and value of quantitative dynamic contrast enhancement MR imaging in the evaluation of sinonasal tumors. Chin Med J (Engl) 2014;127:2259-64. [PubMed]
- Farrar CT, Kamoun WS, Ley CD, et al. Sensitivity of MRI tumor biomarkers to VEGFR inhibitor therapy in an orthotopic mouse glioma model. PLoS One 2011;6:e17228. [PubMed]
- Zahra MA, Hollingsworth KG, Sala E, et al. Dynamic contrast-enhanced MRI as a predictor of tumour response to radiotherapy. Lancet Oncol 2007;8:63-74. [PubMed]
- Semple SI, Harry VN, Parkin DE, et al. A combined pharmacokinetic and radiologic assessment of dynamic contrast-enhanced magnetic resonance imaging predicts response to chemoradiation in locally advanced cervical cancer. Int J Radiat Oncol Biol Phys 2009;75:611-7. [PubMed]
- Liu YH, Ye JM, Xu L, et al. Effectiveness of dynamic contrast-enhanced magnetic resonance imaging in evaluating clinical responses to neoadjuvant chemotherapy in breast cancer. Chin Med J (Engl) 2011;124:194-8. [PubMed]
- Vaupel P. Tumor microenvironmental physiology and its implications for radiation oncology. Semin Radiat Oncol 2004;14:198-206. [PubMed]
- Loncaster JA, Carrington BM, Sykes JR, et al. Prediction of radiotherapy outcome using dynamic contrast enhanced MRI of carcinoma of the cervix. Int J Radiat Oncol Biol Phys 2002;54:759-67. [PubMed]
- George ML, Dzik-Jurasz AS, Padhani AR, et al. Non-invasive methods of assessing angiogenesis and their value in predicting response to treatment in colorectal cancer. Br J Surg 2001;88:1628-36. [PubMed]
- Hawighorst H, Engenhart R, Knopp MV, et al. Intracranial meningeomas: time- and dose-dependent effects of irradiation on tumor microcirculation monitored by dynamic MR imaging. Magn Reson Imaging 1997;15:423-32. [PubMed]
- Nakagawa K, Aoki Y, Akanuma A, et al. Radiation therapy of intracranial germ cell tumors with radiosensitivity assessment. Radiat Med 1992;10:55-61. [PubMed]
- Tofts PS, Brix G, Buckley DL, et al. Estimating kinetic parameters from dynamic contrast-enhanced T(1)-weighted MRI of a diffusable tracer: standardized quantities and symbols. J Magn Reson Imaging 1999;10:223-32. [PubMed]
- Reitan NK, Thuen M, Goa PE, et al. Characterization of tumor microvascular structure and permeability: comparison between magnetic resonance imaging and intravital confocal imaging. J Biomed Opt 2010;15:036004. [PubMed]
- Ferrier MC, Sarin H, Fung SH, et al. Validation of dynamic contrast-enhanced magnetic resonance imaging-derived vascular permeability measurements using quantitative autoradiography in the RG2 rat brain tumor model. Neoplasia 2007;9:546-55. [PubMed]
- Azar JM, Schneider BP, Einhorn LH. Is the blood-brain barrier relevant in metastatic germ cell tumor? Int J Radiat Oncol Biol Phys 2007;69:163-6. [PubMed]
- Aoyama H. Radiation therapy for intracranial germ cell tumors. Prog Neurol Surg 2009;23:96-105. [PubMed]
- Skowrońska-Gardas A. A literature review of the recent radiotherapy clinical trials in pediatric brain tumors. Rev Recent Clin Trials 2009;4:42-55. [PubMed]
- Chen YW, Huang PI, Ho DM, et al. Change in treatment strategy for intracranial germinoma: long-term follow-up experience at a single institute. Cancer 2012;118:2752-62. [PubMed]
- Therasse P, Arbuck SG, Eisenhauer EA, et al. New guidelines to evaluate the response to treatment in solid tumors. European Organization for Research and Treatment of Cancer, National Cancer Institute of the United States, National Cancer Institute of Canada. J Natl Cancer Inst 2000;92:205-16. [PubMed]
- Qu B, Du L, Huang Y, et al. Clinical analysis of intracranial germinoma's craniospinal irradiation using helical tomotherapy. Chin J Cancer Res 2014;26:247-54. [PubMed]