Doublecortin-like kinase 1 exhibits cancer stem cell-like characteristics in a human colon cancer cell line
Introduction
Colorectal cancer is the third most common cancer diagnosed and the third leading cause of cancer-related deaths in both men and women in the United States (1). In the last 20 years, progress in the treatment of colon cancer has improved the quality of life of patients through the use of innovative minimally invasive surgical techniques and multimodality treatments (2). However, the survival of patients with advanced disease has not improved (3). In fact, up to 50% of patients will relapse after surgical resection and ultimately die of metastatic disease (4-7). Although the reason for this is not fully understood, the presence of chemotherapy-resistant cancer stem cells (CSCs) is thought to be one the primary causes for tumor recurrence (8). This small subpopulation of cells has been identified in several solid tumors, including colon cancer. Recent evidence shows that CSCs possess certain characteristics that give them the ability to maintain the tumor population, metastasize, and be resistant to chemoradiotherapy. Thus, the CSC hypothesis has been proposed as a hierarchical model of tumor origin.
Putative CSC populations have been identified in several solid malignancies based on the up-regulation of specific surface markers when cultured under non-adherent conditions in serum-free media. The growth of these spherical colonies is also considered to reflect other stem cell-like features, such as the self-renewal ability (9). Interestingly, investigators have shown that restricted oxygen conditions can increase the CSC fraction and promote acquisition of a stem-like state (10). Recently, doublecortin-like kinase 1 [DCLK1, also known as KIAA0369 (11) and DCAMKL1 (12)], a microtubule-associated kinase expressed in postmitotic neurons, has been identified as a putative intestinal and colon stem cell surface marker (13-16). DCLK1 has been found to be associated with colon cancer (17) as well as other types of adenocarcinoma including esophageal (18) and pancreatic (19). The DCLK1 gene encodes different isoforms through alternative splicing mechanism and alternative promoters (11). DCLK1 functions in the regulation of microtubule polymerization through its doublecortin domain (20,21), and it serves as a serine-threonine protein kinase through its c-terminal protein kinase domain (22). It is involved in normal nervous system development (11,23,24), and it is correlated with general cognition and verbal memory function (25).
In the colon, we have found that DCLK1 positive cells in tumors reside in a niche at the crypt base among the Paneth cells and above the crypt base in an area, which includes the +4 compartment, were quiescent stem cells are thought to reside (13,26). However, currently, there are opposing opinions on the exact identity and functional characteristics of DCLK1 positive cells. Some view them as gastrointestinal stem cells that fuel the self-renewal process (13), whereas others view them as tuft cells (27) or as an enteroendocrine subtype (26).
The purpose of this study was to build on our initial observations of DCLK1 + cells in colon cancer and to identify and characterize the stem-like characteristics of the DCLK1 + cell fraction.
Materials and methods
Cell cultures
Human colon cancer HCT116 cells were a gift from Dr. Prescott Deininger in Tulane Cancer Center. Cells were maintained in McCoy’s 5A medium (Gibco, Grand Island, NY, cat # 16600) supplemented with 10% FBS in 37 °C incubator with 5% CO2.
Spheroid formation
HCT116 cells were dissociated with 0.25% Trypsin-EDTA solution (Sigma-Aldrich, St. Louis, MO) until single cell suspension was obtained. Cells were re-suspended in the serum-free spheroid formation medium, which consists of 100 µg/mL human recombinant epidermal growth factor (EGF, Stemgent, St. Diego CA, USA), 100 µg/mL human recombinant basic fibroblast growth factor (bFGF, Biosource, Grand Island, NY), and 0.5× B27 supplement (Gibco, Grand Island, NY) in the McCoy’s 5A medium. 2×104 cells were plated in the ultra-low attachment 6 well plate (Fisher Scientific) in each well. Fresh medium was added to the cells every 3 days and spheroids were maintained in cultures for 7 days. For the spheroids formed under hypoxia condition, 1% or 5% O2 was applied.
RNA harvest
Total RNA from HCT116 adherent parental cells and spheroids was isolated using the RNeasy Mini Kit (Qiagen, Valencia, CA) according to the manufacturer’s instructions.
Reverse transcription polymerase chain reaction (RT-PCR)
cDNA was generated using the Reverse Transcription System (Promega, Madison, WI) according to the manufacturer’s instruction. Briefly, 1 µg of RNA was reverse transcribed in 20 µL reaction. The first-stand cDNA amplification mixture was diluted to 100 µL with nuclease-free water.
Quantitative real time PCR [(q)RT-PCR]
Gene expression was determined on an iCycler iQ5 fluorescence thermocycler (Bio-Rad Laboratories, Hercules, CA) using SYBR GreenER™ qPCR SuperMix for iCycler® Instrument (Life Technologies, Grand Island, NY, cat #11761-100). (q)RT-PCR was carried out according to the manufacturer’s instruction with some modifications. Briefly, 5 µL cDNA from RT-PCR was added to a 25 µL reaction. Primers for the human DCLK1 are: 5'-TGAAGGGTACGCTCCTCAGT-3' (forward) and 5'-GCTACACTCTGACCGCATGA-3' (reverse). Beta-actin was used as an internal control and the primers are: 5'-GGACTTCGAGCAAGAGATGG-3' (forward) and 5'- AGCACTGTGTTGGCGTACAG-3' (reverse).
Fluorescence-activated cell sorting (FACS)
CD44 is a universal cell surface glycoprotein expressed in a large number of mammalian cell types. It was used as an internal control of DCLK1 in this experiment. Briefly, HCT116 parental cells and spheroid cells were dissociated into single cell with Accutase (Invitrogen, Carlsbad, CA). Cells were suspended in PBS with 10% FBS and incubated with DCAMKL-1 polyclonal rabbit anti-human antibody (Abcam, Cambridge, MA) in dark at room temperature for one hour. After primary DCAMKL-1 antibody incubation cells were washed and incubated with FITC-conjugated goat anti-rabbit secondary antibody (Abcam, Cambridge, MA) in dark at room temperature for 30 minutes. After washing, cells were sorted through a cell sorter (FACS Aria III, BD). The cell line was independently analyzed three times and the mean value ± standard deviation calculated.
Extreme limiting dilution analysis (ELDA)
ELDA was performed according to Hu and Smyth’s approach (28). In brief, HCT116 cells from passage and from spheroids were dissociated into single cell suspension. Cells of 1, 100, 1,000, and 10,000 in 100 µL per well were plated in ultra-low attachment 96 well plate (Fisher Scientific, Corning 3474) with 24 wells for each individual concentration and maintained in the serum-free spheroid formation medium for 5 days. Spheroids were fixed with 4% formalin and number of spheroids was counted under light microscope.
Statistical analysis
All data are shown as mean ± SEM. Statistical analysis between the DCLK1 mRNA and antigen expression in parent and the HCT116 spheroids was performed using the Student’s t-test. The same analysis was applied to analyze the correlation between cell self-renewal capability and cell derivation. P<0.05 was considered to be statistically significant.
Results
DCLK1 mRNA is expressed in HCT116 cells
It has been reported that DCLK1 is expressed in both human normal (26) and colorectal cancer tissue (17). In order to determine whether DCLK1 is expressed in HCT116 cells and its relative expression level compared to beta-actin, a pair of primers that can recognize DCLK1 isoform_1(DCLK-long-B), isoform_2(DCLK-Short-B) and isoform_3(DCLK-Short-A) were used in the (q)RT-PCR experiment. The expression of DCLK1 isoform_4(CARP) was not included. Results indicated that the primers specifically identified DCLK1 isoform_1, 2 and 3 expression in the HCT 116 cells (Figure 1A) and their expression level was very low compared to beta actin, which is 1.92×10–6± 0.96×10–6.
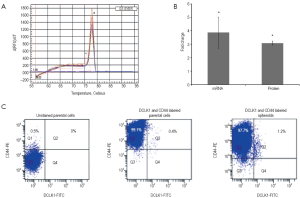
Expression of DCLK1 is up-regulated in HCT116 spheroids both transcriptionally and translationally under normal O2 condition
Cancer stem cells (CSCs) consist of a higher percentage in spheroids (29-31). In order to evaluate DCLK1 expression in the CSCs, spheroids were formed from HCT116 cells under normal O2 condition in serum-free condition. (q)RT-PCR was applied to monitor DCLK1 mRNA expression. Compared to the adherent parental cells, DCLK1 mRNA expression was significantly increased in the spheroids (3.86±1.16 fold; P=0.0026, Figure 1B), which indicates that DCLK1 mRNA is up-regulated in the CSCs. Since DCLK1 is a transmembrane protein (32) and it is expressed on the cell surface (14), FACS was carried out to determine whether DCLK1 is up-regulated on the protein level in spheroids. Results demonstrated that DCLK1 expression in the spheroids keeps the same up-regulation trend as that at the transcriptional level (Figure 1B,C). DCLK1 positive cells in the parental cells consist of 0.5±0.1%, whereas in the spheroid cells, DCLK1 positive cells consisted of 1.4±0.3%. That is 3.1-fold increase and it is statistically significant (P=0.015), which indicates that DCLK1 protein is up-regulated in the CSCs. So in summary, the expression of DCLK1 is increased in HCT116 cell spheroids both transcriptionally and translationally, which indicates that DCLK1 expression in CSCs is up-regulated on both transcription and translation level.
Expression of DCLK1 is up-regulated in HCT116 spheroids both transcriptionally and translationally under hypoxia condition
Hypoxia is a common feature of solid tumors under normal biological condition or during anti-angiogenesis, irradiation and chemotherapy (33). In order to determine whether hypoxia affects expression of DCLK1 in CSCs, HCT116 spheroids were cultured under hypoxia condition (5% O2 for mRNA harvest and 1% O2 for FACS). (q)RT-PCR results showed that DCLK1 mRNA expression was significantly increased in the spheroids (3.4±1.08 fold; P=0.03, Figure 2A) compared to the parental cells, which indicates that DCLK1 mRNA is up-regulated in the CSCs under hypoxia condition. In order to let the cells grow under more extreme hypoxia condition, spheroids were cultured at 1% O2 for the FACS. Results showed that DCLK1 positive cells account for 0.4±0.1% in the parental passage cells; while in the spheroids, DCLK1 positive cells account for 4.3±1.4% (Figure 2A,B). DCLK1 protein expression was increased significantly in the spheroids than the parental passage cells (10.8±0.3 fold; P=0.006), which indicates that DCLK1 protein is up-regulated in the CSCs under hypoxia condition. So in summary, under hypoxia condition, the expression of DCLK1 is increased in HCT116 cell spheroids both transcriptionally and translationally, which indicates that DCLK1 expression in CSCs is up-regulated on both transcription and translation level.
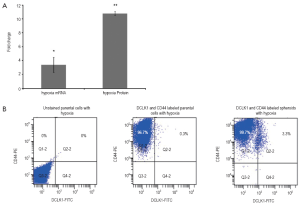
Cells derived from spheroids possess stronger spheroids formation capability
In the CSCs hypothesis, it is believed that CSCs possess similar biological properties as normal stem cells, such as its capability in indefinite self-replication. Through aberrant differentiation, CSCs can generate other cancer cells (34). In order to investigate whether CSCs possess stronger spheroids formation capability, cells from parental HCT116 cells or spheroids were plated at different concentration for spheroid formation according to the ELDA approach. Results demonstrated that cells from spheroids possess stronger spheroid formation capability compared to the parental cells, and this capability is dose dependent (Figure 3). Cells from spheroids at 100 and 1,000 cells/well possess significant stronger spheroids formation capability (P=8.357e-05 and 1.324e-06, respectively). Whereas for the concentration of 1 and 10 cell(s)/well, there is no significant difference was found between the cells derived from either parental or spheroids. This data indicates that CSCs possess the capability of giving rise to other cancer cells, and this capability is significantly stronger than parental cells. Up-regulation of DCLK1 in the CSCs may be correlated with the stronger cell proliferation capability.
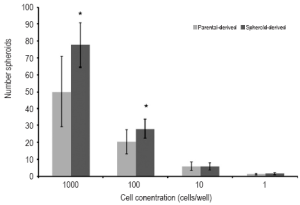
Discussion
In this report, we present evidence that one of the best-known human cancer cell lines derived from colorectal adenocarcinomas contains a subpopulation of DCLK1 + cells with stem-like properties as demonstrated by their up-regulation when cultivated under stem-cell-selective conditions. Under these conditions, we also demonstrated that DCLK1 + cells exhibited other key biological properties of CSCs, including self-renewal and proliferation.
Serum-free culture conditions supplemented with growth factors have been successfully applied to cultivate cells with stem-like properties from several malignancies (31,35-37). In this study, we applied a serum-free culture condition to cultivate CSCs from a colon cancer cell line. Tumor sphere formation was observed within 8 weeks, could be sustained in culture for more than 5 months, and retained the expression of well-known cell surface markers, such as CD44. As controls, adherent primary cultures were also generated under traditional culture conditions using a serum-containing medium. Interestingly, DCLK1 + cells were found to comprise only a small fraction of the total tumor population in our adherent primary cultures of HCT116 human colon cancer cells. However, consistent with stem like properties, the percentage of DCLK1 + cells significantly increased in the tumor sphere-forming colonies. Considering the level of DCLK1 expression observed in our colon cancer cells and the belief that CSCs are only a small sub-population of tumor specimens, our results suggest that DCLK1 may be a marker expressed on functional CSCs.
High level of DCLK1 expression has been observed in other gastrointestinal tumors/cancers as well. Vega and colleagues (18) reported that DCLK1 increased significantly in both Barrett’s esophagus with/without dysplasia and esophageal adenocarcinoma when compared to the normal esophageal mucosa. They also observed DCLK1 was progressively increased during the progression from Barrett’s esophagus with dysplasia to esophageal adenocarcinoma. It was also reported that in the Hepatitis C virus-induced liver carcinogenesis, DCLK1 was highly expressed and DCAMKL-1-positive cells isolated from hepatoma cell lines possess the capability to form spheroids (38). Sureban and colleagues found that DCLK1 was increased in both mouse pancreatic cancer model and human pancreatic adenocarcinoma (19). Taken together, these observations suggest that the up-regulation of DCLK1 plays a critical role in the development of carcinogenesis.
Whether DCLK1 can be used as an additional marker to further define the CSC population in colorectal cancer remains a topic of debate. May and colleagues (13,14) identified DCLK1 as a novel gastrointestinal stem cell and adenoma stem cell marker following radiation injury and in adenomatous polyposis coli (APC)/multiple intestinal neoplasia mice. They observed that DCLK1 positive cells are located at the +4 position, just above Paneth cells, and co-localized with some musashi-1 (MSI-1), a well-accepted stem cell marker. They further identified that DCLK1 was a quiescent intestinal stem cell marker, and it can be used to distinguish quiescent stem cells from the cycling stem/progenitors. However, Gerbe and colleagues (39) proposed that DCLK1 is not a marker for intestinal epithelial stem cells, but a highly specific and robust marker for post-mitotic, differentiated, tuft cells, which is a minority cell lineage of the intestinal epithelium. Using DCLK1 immunohistochemistry staining in normal colorectal biopsies from 14 patients, our lab identified that expression of DCLK1 was not confined to the stem cell compartment, with 70% of DCLK-1 positive cells located in the lower third of the crypt, 26% in the middle third and 4% in the upper third. We also identified that DCLK1 only co-localized with leucine-rich-repeat-containing G-protein-coupled receptor 5 (Lgr5), an intestinal stem cell marker, at the crypt base, but co-stained with chromogranin-A (CgA), an enteroendocrine cell marker, throughout the crypt. So we believe that DCLK1 marks a subset of colorectal stem cells, as well as a subset of enteroendocrine cells (26). The most recent publication from Nakanishi and colleagues reported that DCLK1 is only expressed in the tumor stem cells but not in the normal stem cells, so it is a unique intestine tumor stem cell marker (15).
Using DCLK1 as a target for tumor/cancer therapy has gained promising progress in research. Sureban and colleagues (40,41) reported that growth of HCT116 xenografts in nude mice was arrested after DCLK1 knockdown by siRNA. Verissimo and colleagues observed that apoptosis was induced in neuroblastoma cells after DCLK1 knockdown (42). Nakanishi and colleagues investigated whether targeting DCLK1 marked tumor stem cells can be an effective approach to treat tumor. They found that diphtheria toxin only cause apoptosis in the DCLK1 positive tumor stem cells, but not in the DCLK1 negative cells, and interruption of the tumor progeny supply by DCLK1-positive TSCs can cause tumor regression during turnover of polyps (15). So targeting DCLK1, either directly or indirectly, might be an effective approach for the treatment of tumors/cancers.
Mechanism of how DCLK1 affect tumor genesis still remains unclear. Sureban and colleagues (19,40,41) reported that in the HCT116 xenografts or pancreatic cancer cells after DCLK1 siRNA treatment, the antitumor microRNA let-7a and miR-144 were up-regulated, and their downstream proto-oncogene c-Myc and Notch-1 were down-regulated, respectively. What’s more, they also observed that the epithelial mesenchymal transition (EMT) inhibitor miR-200a was up-regulated and the EMT-associated transcription factors ZEB1, ZEB2, Snail and Slug were down-regulated. So they believe that the direct regulatory links between DCAMKL-1, microRNAs, and EMT might enable DCLK1 to become a novel target for tumor/cancer therapy.
Oxygen tension is tightly regulated under homeostasis condition. Disorder of oxygenation levels can result in severe diseases. About 70 years ago, Warburg had realized that low oxygenation levels (now termed as hypoxia) is the prime cause of cancer (43,44). Hypoxia is a common fundamental feature of solid tumors under normal biological condition or during anti-angiogenesis, irradiation and chemotherapy (33). Hypoxia affects tumor progression directly or in-directly, and many molecular pathways and genes are involved, including hypoxia induced factor-1 (HIF-1), VEGF, etc. It has been identified that low oxygen tension associated with maintenance of an undifferentiated cell state (45,46). The response of stem cells (SC) to hypoxia is one of the main mechanisms of an organism’s adaptation to changing terms of external and internal environment. Hypoxia is likely to be a functional component of a normal stem cell niche. Interestingly, hypoxia promotes the self-renewal of embryonic stem (ES) cells and has been shown to regulate cancer stem cells and promotes their self-renewal capability (45,47,48). Indeed, investigators have shown that restricted oxygen conditions increase the CSC fraction and promote acquisition of a stem-like state. Lin and colleagues (10) observed that CD133 + enriched colorectal CSCs can survive under hypoxia and serum depletion condition. Long-term hypoxia condition significantly enhanced HCT116 xenografts growth rate, increased HIF-1 expression, and increased microvessel density in the tumor tissue (49). Our results demonstrated that under hypoxia condition, DCLK1 expression was significantly increased in the HCT116 spheroids transcriptionally and translationally. To our knowledge, this is the first time that DCLK1 expression was investigated under hypoxia condition in the colorectal cancer cells. Whether the increase of DCLK1 is correlated with hypoxia-induced tumor angiogenesis, increasing mutation rate, metastasis and resistance to radiation and chemotherapy needs further investigation.
Two important characteristics of stem cells are its self-replication and asymmetric cell division capability. CSCs are hypothesized to possess those stem-like properties and that’s why radio-chemoresistance exists. In our experiment, we observed that cells generated from HCT116 spheroids possess significantly stronger self-renewal capability compared to the parental adhesion HCT116 cells, and it is cell dose-dependent. This may be due to the higher percentage of CSCs in the spheroids. So our results indicate that CSCs are capable for self-renewal. To target the CSCs for tumor therapy will be an effective approach for tumor therapy.
In summary, we demonstrated that DCLK1 expression was up-regulated in the HCT116 spheroids under both normal and hypoxia condition. Cells from spheroids are more capable to produce progenies. Our data also indicates that DCLK1 is up-regulated in the CSCs, and this may be correlated with their stronger self-renewal capability. DCLK1 may become a novel target for colorectal cancer therapy. Further studies from patients’ specimens are needed for a continued clarification of the role of these cells in therapy response and therefore to contribute for the establishment of novel therapeutic strategies.
Acknowledgements
We’d like to thank The Louisiana Cancer Research Consortium FACS Core for providing the FACS service.
Disclosure: The authors declare no conflict of interest.
References
- Available online: http://www.cancer.org/acs/groups/cid/documents/webcontent/003096-pdf.pdf
- American Cancer Society. Colorectal Cancer Facts & Figures. Atlanta, Georgia: American Cancer Society, 2011-2013.
- Welch JP, Donaldson GA. The clinical correlation of an autopsy study of recurrent colorectal cancer. Ann Surg 1979;189:496-502.
- De Dosso S, Sessa C, Saletti P. Adjuvant therapy for colon cancer: present and perspectives. Cancer Treat Rev 2009;35:160-6.
- Kopetz S, Freitas D, Calabrich AF, et al. Adjuvant chemotherapy for stage II colon cancer. Oncology (Williston Park) 2008;22:260-70; discussion 270, 273, 275.
- Marshall JL, Haller DG, de Gramont A, et al. Adjuvant Therapy for Stage II and III Colon Cancer: Consensus Report of the International Society of Gastrointestinal Oncology. Gastrointest Cancer Res 2007;1:146-54.
- de Gramont A, Boni C, Navarro M, et al. Oxaliplatin/5FU/LV in the adjuvant treatment of stage II and stage III colon cancer: Efficacy results with a median follow-up of 4 years. J Clin Oncol 2005;23:abstr 3501.
- Dean M, Fojo T, Bates S. Tumour stem cells and drug resistance. Nat Rev Cancer 2005;5:275-84.
- Reya T, Morrison SJ, Clarke MF, et al. Stem cells, cancer, and cancer stem cells. Nature 2001;414:105-11.
- Lin SP, Lee YT, Wang JY, et al. Survival of cancer stem cells under hypoxia and serum depletion via decrease in PP2A activity and activation of p38-MAPKAPK2-Hsp27. PLoS One 2012;7:e49605.
- Omori Y, Suzuki M, Ozaki K, et al. Expression and chromosomal localization of KIAA0369, a putative kinase structurally related to Doublecortin. J Hum Genet 1998;43:169-77.
- Matsumoto N, Pilz DT, Ledbetter DH. Genomic structure, chromosomal mapping, and expression pattern of human DCAMKL1 (KIAA0369), a homologue of DCX (XLIS). Genomics 1999;56:179-83.
- May R, Riehl TE, Hunt C, et al. Identification of a novel putative gastrointestinal stem cell and adenoma stem cell marker, doublecortin and CaM kinase-like-1, following radiation injury and in adenomatous polyposis coli/multiple intestinal neoplasia mice. Stem Cells 2008;26:630-7.
- May R, Sureban SM, Hoang N, et al. Doublecortin and CaM kinase-like-1 and leucine-rich-repeat-containing G-protein-coupled receptor mark quiescent and cycling intestinal stem cells, respectively. Stem Cells 2009;27:2571-9.
- Nakanishi Y, Seno H, Fukuoka A, et al. Dclk1 distinguishes between tumor and normal stem cells in the intestine. Nat Genet 2013;45:98-103.
- Giannakis M, Stappenbeck TS, Mills JC, et al. Molecular properties of adult mouse gastric and intestinal epithelial progenitors in their niches. J Biol Chem 2006;281:11292-300.
- Gagliardi G, Goswami M, Passera R, et al. DCLK1 immunoreactivity in colorectal neoplasia. Clin Exp Gastroenterol 2012;5:35-42.
- Vega KJ, May R, Sureban SM, et al. Identification of the putative intestinal stem cell marker doublecortin and CaM kinase-like-1 in Barrett’s esophagus and esophageal adenocarcinoma. J Gastroenterol Hepatol 2012;27:773-80.
- Sureban SM, May R, Lightfoot SA, et al. DCAMKL-1 regulates epithelial-mesenchymal transition in human pancreatic cells through a miR-200a-dependent mechanism. Cancer Res 2011;71:2328-38.
- Lin PT, Gleeson JG, Corbo JC, et al. DCAMKL1 encodes a protein kinase with homology to doublecortin that regulates microtubule polymerization. J Neurosci 2000;20:9152-61.
- Kim MH, Cierpicki T, Derewenda U, et al. The DCX-domain tandems of doublecortin and doublecortin-like kinase. Nat Struct Biol 2003;10:324-33.
- Shang L, Kwon YG, Nandy S, et al. Catalytic and regulatory domains of doublecortin kinase-1. Biochemistry 2003;42:2185-94.
- Burgess HA, Martinez S, Reiner O. KIAA0369, doublecortin-like kinase, is expressed during brain development. J Neurosci Res 1999;58:567-75.
- Mizuguchi M, Qin J, Yamada M, et al. High expression of doublecortin and KIAA0369 protein in fetal brain suggests their specific role in neuronal migration. Am J Pathol 1999;155:1713-21.
- Le Hellard S, Håvik B, Espeseth T, et al. Variants in doublecortin- and calmodulin kinase like 1, a gene up-regulated by BDNF, are associated with memory and general cognitive abilities. PLoS One 2009;4:e7534.
- Gagliardi G, Moroz K, Bellows CF. Immunolocalization of DCAMKL-1, a putative intestinal stem cell marker, in normal colonic tissue. Pathol Res Pract 2012;208:475-9.
- Saqui-Salces M, Keeley TM, Grosse AS, et al. Gastric tuft cells express DCLK1 and are expanded in hyperplasia. Histochem Cell Biol 2011;136:191-204.
- Hu Y, Smyth GK. ELDA: extreme limiting dilution analysis for comparing depleted and enriched populations in stem cell and other assays. J Immunol Methods 2009;347:70-8.
- Kirkland SC, Ying H. Alpha2beta1 integrin regulates lineage commitment in multipotent human colorectal cancer cells. J Biol Chem 2008;283:27612-9.
- Ponti D, Costa A, Zaffaroni N, et al. Isolation and in vitro propagation of tumorigenic breast cancer cells with stem/progenitor cell properties. Cancer Res 2005;65:5506-11.
- Zhong Y, Guan K, Guo S, et al. Spheres derived from the human SK-RC-42 renal cell carcinoma cell line are enriched in cancer stem cells. Cancer Lett 2010;299:150-60.
- Sossey-Alaoui K, Srivastava AK. DCAMKL1, a brain-specific transmembrane protein on 13q12.3 that is similar to doublecortin (DCX). Genomics 1999;56:121-6.
- Harrison L, Blackwell K. Hypoxia and anemia: factors in decreased sensitivity to radiation therapy and chemotherapy? Oncologist 2004;9:31-40.
- Song LL, Miele L. Cancer stem cells--an old idea that's new again: implications for the diagnosis and treatment of breast cancer. Expert Opin Biol Ther 2007;7:431-8.
- Cao L, Zhou Y, Zhai B, et al. Sphere-forming cell subpopulations with cancer stem cell properties in human hepatoma cell lines. BMC Gastroenterol 2011;11:71.
- Fan X, Liu S, Su F, et al. Effective enrichment of prostate cancer stem cells from spheres in a suspension culture system. Urol Oncol 2012;30:314-8.
- Wang Y, Yu Y, Tsuyada A, et al. Transforming growth factor-β regulates the sphere-initiating stem cell-like feature in breast cancer through miRNA-181 and ATM. Oncogene 2011;30:1470-80.
- Ali N, Allam H, May R, et al. Hepatitis C virus-induced cancer stem cell-like signatures in cell culture and murine tumor xenografts. J Virol 2011;85:12292-303.
- Gerbe F, Brulin B, Makrini L, et al. DCAMKL-1 expression identifies Tuft cells rather than stem cells in the adult mouse intestinal epithelium. Gastroenterology 2009;137:2179-80; author reply 2180-1.
- Sureban SM, May R, Ramalingam S, et al. Selective blockade of DCAMKL-1 results in tumor growth arrest by a Let-7a MicroRNA-dependent mechanism. Gastroenterology 2009;137:649-59, 659.e1-2.
- Sureban SM, May R, Mondalek FG, et al. Nanoparticle-based delivery of siDCAMKL-1 increases microRNA-144 and inhibits colorectal cancer tumor growth via a Notch-1 dependent mechanism. J Nanobiotechnology 2011;9:40.
- Verissimo CS, Molenaar JJ, Meerman J, et al. Silencing of the microtubule-associated proteins doublecortin-like and doublecortin-like kinase-long induces apoptosis in neuroblastoma cells. Endocr Relat Cancer 2010;17:399-414.
- Warburg O. On respiratory impairment in cancer cells. Science 1956;124:269-70.
- Warburg O. On the origin of cancer cells. Science 1956;123:309-14.
- Rajan P, Panchision DM, Newell LF, et al. BMPs signal alternately through a SMAD or FRAP-STAT pathway to regulate fate choice in CNS stem cells. J Cell Biol 2003;161:911-21.
- Yun Z, Maecker HL, Johnson RS, et al. Inhibition of PPAR gamma 2 gene expression by the HIF-1-regulated gene DEC1/Stra13: a mechanism for regulation of adipogenesis by hypoxia. Dev Cell 2002;2:331-41.
- Studer L, Csete M, Lee SH, et al. Enhanced proliferation, survival, and dopaminergic differentiation of CNS precursors in lowered oxygen. J Neurosci 2000;20:7377-83.
- Morrison SJ, Csete M, Groves AK, et al. Culture in reduced levels of oxygen promotes clonogenic sympathoadrenal differentiation by isolated neural crest stem cells. J Neurosci 2000;20:7370-6.
- Yu L, Hales CA. Long-term exposure to hypoxia inhibits tumor progression of lung cancer in rats and mice. BMC Cancer 2011;11:331.